tRNAHis/GUG识别特性的分子力学模拟
作者:姚立新 曹槐 刘次全
单位:姚立新(广东药学院药物研究所,广东广州 510224);曹槐(云南大学现代生物学研究中心);刘次全(中国科学院昆明动物研究所细胞与分子进化重点实验室)
关键词:计算机辅助分子建模(CAMM);识别特性;tRNAHis;GUG;分子力学;氢键网络
广东药学院学报000101 摘 要 本文选用经过实验验证的碱基序列,用简化的方式,构建了被水分子和镁离子修饰的 核酸序列的分子模型,应用分子力学模拟方法对序列进行能量优化,对优化后序列的构象参 数、成键状况和能量数据等进行了分析。对tRNAHHis/GUG的识别特性作了初步的探索 ,得到 了和实验结果相近的结论。此外,还从能力学的角度讨论了溶剂-溶质-溶剂相互作用形成 的网状氢键网络对核酸结构稳定性的影响,探讨了非Crick-Watson G U、U U配对的能力学 特征并存在于被水分子和镁离子修饰的核酸序列中的G U、U U配对情况。
, http://www.100md.com
中图号 P969.1 文献标识码:A 文章编号:1006-8783(2000)01-0001-06
Molecular Mechanics Simulation of Recognition
Identity of tRNAHis/ GUG
YAO Li-xin
(Institute of Materia Medica, Guangdong College of Pharmacy, Guangzhou,510 240)
CAO Huai
(Modern Biological Research Center, Yunnan University)
, 百拇医药
LIU Ci-quan
(Key Laboratory of Cellular and Molecular Evolution, Kunming Institute of Zo ology, Chinese Academy of Sciences)
Abstract A group of nucleic acid sequences retrieved from experiment was analyzed based o n molecular mechanics simulation method to prove tRNA recognition identity that was derived from parts of tRNAHis/GUG sequence. A simplified molecular mod el of nucleic acid sequences with cage-like water molecules and Mg2+ ions was co nstruc ted. Based upon the primary analyses of the optimized molecular models, such as conformation parameters, bonding propensities, and energies of optimized models, etc., the recognition identity of tRNAHis/GUG was probed and the result w as s imilar to experiment-determined ones. Some problems, such as stem-loop structure (hair-pin structure), the impacts of charged metal ions (Mg2+) and H2O molecule s on the stability of nucleic acid sequences, non-Watson-Crick base pairing (i.e . U.U/G.U base pairing), and stabilizing influence of the ″cage-like″ solven t-solvent and solvent-solute bonds were also discussed.
, http://www.100md.com
Key Words computer-aided molecular modeling (CAMM), tRNAHis/GUG , recognition identity, molecular mechanics, hydrogen-bond network
CLC number R 969.1 Document co de:A Article ID:1006-8783(2000)01-0001-06
1 Introduction
The interaction of mRNA with tRNA charged with amino acid takes part in the determination of collinear genetic code. The charging of amino acids with tRNAs is catalyzed by aminoacyl-tRNA synthetases (aaRs)[1].The nucleic acid sequences of tRNAs and minor variations both in nucleic sequ ences and the conformations are concerned with the interaction of tRNAs with ami noacyl-tRNA synthetases, both structurally and functionally[2].So far, 20 kinds of aminoacyl-tRNA synthetases have been reported,each of them responsible for the relevant amino acid and the special tRNA. Usually, each enzym e can be charged with various iso-acceptors of different tRNA molecules[1 ]. Analogous to RNA replicase that has been considered as the first ribosome in the evolution [2], the occurrence of aminoacyl-tRNA synthetases in early protein evolution seems i mproving fidelity of protein synthesis by the special charging with tRNA. Due to the universal conservation of aminoacyl-tRNA synthetases, it has been considere d that the enzymes had come into being in the early evolution, playing the same important role as the catalysis effect that RNAs did in the soup of early organi sm system, the recognition identity between aminoacyl-tRNA and tRNA led to the s peedy evolution of genetic code. This recognition system was called second genet ic code or paracoden[3].
, 百拇医药
The recognition of tRNA is so complex that it is not enough to be determined onl y based on clove structure of tRNA4,5. The explication of recognition identity of tRNAs lies i n the comprehensive studies of tertiary structures of tRNAs and various recognit ion elements in various levels, particularly the involved regions for the recogn ition of aminoacyl-tRNA synthetases and tRNAs. Aminoacyl-tRNA synthetases bind t he inner side of L-shaped tRNAs, the binding locus include accepting arm, DHU-ar m and anticodon arm[6]. Traditional codon-anticodon interactions in ribo somes ar e involved with up-stream factors, down-stream factors and RFs (releasing factor s), while translation process in ribosomes is related with interactions in A-si te, P-site and E-site, etc[7]. While keeping the nucleic acids the same in the a nticodon, mutagenesis of 12 nucleic acids in other regions had changed tRNAl eu t o tRNASer[8]. The 0.29nm crystal structure of T. Thermophilus Seryl-tRNA synthetas es complexed with tRNASer showed that, the anti-parallel super-coiling dom ain in one sub-unit of the aminoacyl-tRNA synthetase contacted with TψC loop and vari able pocket and lead accepting arm of tRNA to the active site of the enzyme [9]. T he recognition identity mainly lay in the contact of backbone of tRNA rather tha n in the sequence identity of tRNA[9]. In vitro nucleic synthesis experi ment als o showed that the changing of charging identity only happened in the mutation of G3.U70 base pair in various mutants of inhibitory E. Coli. tRNAs and relat ive a minoacyl-tRNA synthetases carrying tRNAAla/Glu[10]. Evolutionarily , this nondeg enerate paracodon may be more ancient and more decisive, the charging of amino a cids with tRNAs catalyzed by aminoacyl-tRNA synthetases can be considered as the interactive and indirect charging of two different kinds of genetic languages, and ″entries of genetic″ dictionary are carried by tRNAs and aminoacyl-tRNA sy nt hetases[3]. However, for most tRNAs, the recognition identities are main ly relat ed with nucleic acid sequence of anticodons[2、6、11、12], the clarific ation of this paracodon will contribute to further understanding of the evolution of tran slation process in the transmission of genetic information[6].
, http://www.100md.com
CPK (Corey-Pauling-Koltum) modeling and lock-key relationship has been employe d in the stacking structure of tRNA[13], however, it seems inappropriate to use rigid lock-key relationship to describe the flexible interaction of nuc leic acid with proteins. Various methods have been employed in the simulation or theoretical calculation of the recognition identity of tRNA[14、15].Us ing simp lified method to analyze may be possible, such as energy minimization and normal mode methods[16、17]. Based on four sequence retrieved from the concern ed experi ment[18], computer-aided molecular modeling, molecular mechanics methods were performed in this study by using simplified methods.2 Computational Methods
, http://www.100md.com
All the modeling, visualization, simulation, and analyses in this study were done using Biosym/Molecular Simulation Insight-Ⅱ software in SiliconGr aphics Indigo2 workstation (Mountain View, CA 94043-1389). The measuremen t of dihedral angles of the sequences was conducted with Tripos Sybyl molecular modeling software.
3 Molecular Modeling
The stem region of the retrieved[18]sequences was constructed as A-RNA d uplex, and the loop region was constructed as single A-RNA helix. Consideri ng there are Mg2+ ions and H2O molecules in the physiological environmen ts of tRNA, molecular models Mg2+ and H2O molecul es were constructed, while neuridine molecules and sodium ions which usually bin d to tRNAs were omitted due to the restriction of the processing capacity of the software package used. The interaction models of metal ions (Mg2+) and H 2O molecule s with single nucleic acids of A, U, G and C[19] gotten from Laser-Rama n spectroscopy were constructed and then employed in the construction of the nuc leic acid chains (See Fig. 1). The cap of metal ions and water molecules were th en merged or docked to nucleic acid chains (See Fig. 2) separately.
, http://www.100md.com
4 Force Field Selection
ESFF force field from Discover 2.9.7/95.0/3.0.0 was selected in the simulati on. The atomic parameters used in this force field were obtained from experiment s instead of adaptation of ab initio calculation and experimental data[20]. The coverage of ESFF force field is particularly useful for the simulation of o rganic compounds and organi c-metal mixtures [21].
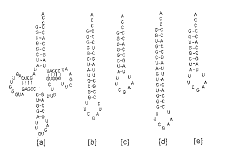
, 百拇医药
Fig. 1 Model of the active interaction between positive ion Mg( H2O)62+and N7 of purines. (From T. Theophanides, 1984) (Hydrogen bonds are showed with dashed lin es)
Fig. 2 Secondary structure of E. coli (G1.C73)tRNAHis/GUG([a]), (G 1.C33)Miniheli xAla([b]), (G1.C23)MicrohelixAla([c]), MinihelixHis([d ]) and ( G1.C73)MicrohelixHis([e]) (from Christopher Francklyn and Paul Schimmel , 1990)
, http://www.100md.com
5 Energy Calculation and Structure Optimization
After the construction of initial structures of complexes with Mg2+ ions and H2O molecules, the whole structures were th en refined and minimized by using steepest descent, followed by conjugate gradie nt, Newton-Rapson, and iterative Newton-Rapson methods automatically and alterna tively till minimum energies were reached. The energy was convergent and root-me an-square (rms) energy gradient of 0.01 kcal/mol ?2 was reached. The de fault charg es from Discover 2.9.7/95.0/3.0.0 package were employed throughout. Four con trol s with the nucleic chains of the same nucleic acid sequences without Mg2+ ions and H2O molecules were also optimized under the same force field and same conditi ons.
, 百拇医药
6 Result and Discussion
6.1 Energy Optimization Seven energy terms were obtained after energy optimization (See table 1).
Tab. 1 Energy terms of the sequences after energy optimizatio n kcal/mol
Total potentialenergy
Internal
Bond
Angle
Torsion
, http://www.100md.com
Out-of
-p lane
Non-
bond
Vdw
vdw-
repulsive
vdw-
dispersive
Electrostati c
MinihelixHis
571.64
, http://www.100md.com
1139.54
108 .09
6 94.87
318.85
17.74
-567.90
576.21
4129.72
-3553.52
-1155.11
MicroehlixHis
-204.07
, 百拇医药
814.77
73.95
486.63
240.01
14.18
1018.84
418.73
3170.23
-2571.50
-1437.57
MinihelixAla
12.42
, 百拇医药
1091.37
96.74
670.84
310.65
13. 14
-1078.95
536.82
4068.25
-3531.43
-1615.78
MicrohelixHis
-837.49
, 百拇医药
774.46
64.74
470.77
226.63
1 2.31
-1611.94
366.55
3098.58
-2732.03
-1978.49
Control.
4891.14
, 百拇医药
778.63
44
443.73
27.172
18.87
4112.4 9
41.77
1771.48
-1729.71
4070.72
.Note: the control sequence was the same as that of MicrohelixAla, but without Mg2+ ions and H2O molecules.
, http://www.100md.com
Analyzed energetically,it is reasonable that the optimum energies of Mini helixHis and MinihelixAla were larger than those of MicrohelixHi s and Microehli xAla. Although the system of sequ ences with Mg2+ ions and H2O molecules were much more complex than those without Mg2+ ions and H2O molecules, the energy of the sequences with Mg2+ ions and H2O molecules were much lower than those of sequences without Mg2+ ions and H 2O mol ecules, partially due to the forming of cage-like solvent-solvent and solvent-so lute hydrogen-bond network and interaction with metal ions. The visualization in the optimization process also proved,the nucleic acid chains without Mg2+ ions and H2O molecules di sbanded much easier. The transitory ″cage-like″ hydrogen bond network between solvent- so lvent and solvent-solute bonds can provide extra stationary factors and reduce t orsion resonance between glycosidic bonds[22], similar to the polyelectr olytic c haracteristics of DNA under ionic environment, and the improvement of dissociati on temperature of DNA caused by the increasing of positive metal ions since meta l io ns had stabilizing effects on nucleic acid molecules[23]. After energy optimiza tion, the Mg2+ ions were mainly distributed in the groove of the nucleic a cid seq uences, in accordance with high salt model (Pack, 1982)[24] and similar to the ion distribution in rRNA[25].
, 百拇医药
It′s noticed that there were G.U and U.U wobble base-pairs (or non-Watson-C r ick base pairs) in MinihelixHis and MinihelixAla sequences. We calcu lated the G.U and U.U base pairs with molecular mechanics using Amber force fi eld, it was found that the increasing variation of hydrogen bond energy term of U.U base pair is larger than that of G.U base pair. Since Hoogsteen hydrogen b onds could be formed between wobble base pairs, the hydrogen bond energy became larger than those of Watson-Crick hydrogen-bonds, while the propensity of U.U b ase pair was smaller than that of G.U base pair. Observed in the final structur e after optimization, there was no hydrogen bond between two wobble uracil pyrom idines, however, there were H3-O2 hydrogen bonds which formed pyromidine rin gs in the U.U wobble pairing. There were also some changes in the hyd rogen bonds close to the wobble pairs. No hydrogen bond was formed in U.A p air above the U.U wobble pairing, while hydrogen bonds different from Watson-Cr i ck pairing appeared in G.C pair that was just below U.U pair. The plane betwee n purine and pyromidine rings was twisted compared with that in Watson-Crick pair. (G)N4-H…N3(C) hydrogen bond was formed in the G C pair. The hydrogen bond bet ween purine ring of G and pyromidine of C, (G)N1-H1…O3(C) was different w ith t hose formed in normal Watson-Crick pairs, i.e. (G)N1-H1…O3(C), (G)N1-H …N3(C) and (G) N2-H…O2(C).
, 百拇医药
In addition, the co-planety in G.U was also poor without hydrogen bond betwee n them, while (G)N3-H2…O2(C), (G)N1-H1…N3(C) hydrogen bonds were fo rmed in G.C pair just above G.U wobble pair. Between G in G.U wobble pair and G in G.C pa ir just below the wobble one, (G in the wobble pair) N2-H…O6(C) hydrogen bo nd was formed. Hydrogen bonds different from Watson-Crick pair, i.e. (G)O6-H…N 3(C ) and (G)N1-H…N3(C) hydrogen bonds were formed in G.C pair just below G.U wobble pair.
, http://www.100md.com
The dihedral angles of the four optimized nucleic acid chains were measured (See table 2.). It can be concluded that the charging of four nucleic acid cha ins (i.e. MinihelixHis, MicrohelixHis, MinihelixAla and Microh elixAla) with amin oacyl-tRNA synthetase is not determined by the whole conformation of the nucleic chains,it is closely related with conformations in some special regions since it seems that no similarity exist between the four nucleic acid chains ju dged only from measured results.
, 百拇医药
Tab. 2 Average diheral angles of the four optimized
nucleic acid chains
MinihelixHis
MicrohelixHis
MinihelixAla
Microheli xAla
α
186.25
227.47
, 百拇医药 -157.28
-119.49
β
-181.75
182.65
-175.37
-179.02
γ
150.14
-242.31
-107.79
111.04
δ
, 百拇医药
89.78
87.91
82.11
74.35
ε
-162.87
-158.58
-177.91
-152.02
ζ
-85.58
-80.84
-68.47
, 百拇医药
-75.29
χ
-131.60
-139.79
218.06
210.39
υ0
-7.92
-0.58
-8.56
0.61
υ1
-7.94
, 百拇医药
-15.33
-9.01
-22.57
υ2
19.37
24.35
21.58
34.4
All of the four sequences in this simulation were composed of stem-loop or hairpin structure. It has been proposed that loop structure p lays an important role in the process of genetic information flow[4]. The seq uences in the stem regions of MinihelixAla and MicrohelixAla were no t the same , however, the average dihedral angles in loop regions of the two sequences mea sured were almost equivalent (See Table 3 and Table 4).Tab. 3 Average angles in the stem regions of
, 百拇医药
the fo ur optimized nucleic acid chains
MinihelixHis
MicrohelixHis
MinihelixAla
Microheli xHis
α
194.73
24.361
-142.82
-105.48
, 百拇医药
β
-176.33
182.67
-175.57
-179.69
γ
148.25
-249.61
-99.36
101.69
δ
93.09
91.59
, 百拇医药
84.59
79.15
ε
-162.55
-153.44
-176.14
-150.11
ξ
-95.14
-86.76
-71.29
-80.67
χ
, 百拇医药
-128.78
-142.01
205.44
210.50
υ0
-4.75
-0.61
-8.40
-1.49
υ1
-8.75
-12.98
-9.25
, 百拇医药
-19.87
υ2
17.60
20.74
21.85
32.15
The conservative G1.C73 base pair had been proved necessary experimentally in the charging with aminoacyl-tRNA synthetase and tRNAAla. No Watson-Crick h ydroge n bond formed between G1.C33, G1.C23 base pairs in MinihelixHis (G1.C33 ), Microh elixHis (G1.C23), MinihelixAla (G1.C33), and MicrohelixAla ( G1.C23)(see Table 5),the same as that in C1.G73 base pair in tRNAAla of Saccaromyces cerevisiae from Brookhaven Protein Databank (PDB). While a non-Watson-Cr ick hydrogen bond w as formed between G1.C73 base pair, some hydrogen bonds were formed between non-pairing bases. The result is in accordance with what has been proved experim entally. And the 2.8 X-ray crystalloid structure of tertiary complexes of tRNAGln, aminoacyl-tRNA synthetase and ATP had proved that the structur e of tRNA in c omplexes was not the same as that of free tRNA, where hydrogen bond between U1 A72 base pair was broken, and a new hairpin structure was formed in CCA of 3′- e nd, which was sunken into a pocket of the enzyme[26]. It seems that non- Watson-C rick hydrogen bonds in G1.C33 and G1.23 base pairs are unstable, and thus lik ely to be broken.Tab. 4 Average angles in the stem regions of
, 百拇医药
the four optimized nucleic acid chains
MinihelixHis
MicrohelixHis
MinihelixAla
Microh elixHis
α
175.90
151.60
188.17
188.17
, http://www.100md.com
β
-177.39
159.26
182.71
182.78
γ
137.51
146.30
136.23
136.42
δ
77.93
78.67
, 百拇医药
59.79
59.69
ε
190.58
190.82
-162.72
-162.79
ζ
-50.47
-46.08
-60.75
-60.34
χ
, http://www.100md.com
-146.81
-138.17
-148.25
-148.26
υ0
-0.48
-14.66
6.45
6.31
υ1
-21.70
-9.32
-29.97
, http://www.100md.com
-29.90
υ2
34.13
27.18
40.55
40.56
Tab. 5 Hydrogen bonds in conservative G1.C23 and G1.C33 base pairs in MinihelixHis,MicrohelixHis, MinihelixAla, and MicrohelixAla.
Hydrogen bonds formed among G1 C23 or G1 C33 base pairs and nearby bases
, http://www.100md.com
MinihelixHis
No hydrogen bond formed in G1 C33 bas e pair and the plane in G1 C33 base pair was twisted
MicrohelixHis
(G1)O6…H-N4(C23) hydrogen bond was formed; and (G2)N3…H-N4(C22) and (G1)N2…H-N4(C23) hy drogen bonds were form ed between G1 and C72 bases
, http://www.100md.com
MinihelixAla
No hydrogen bond formed in G1 C33 base pair and the plane in G1 C33 base pair was twisted
MicrohelixAla
(G1)O6…H-N4(C33) hydrogen bond bet ween G1.C33 base pair and (G2)N2-H…O2(C33) hydrogen bond between G2.C33 base pair were formed.
, http://www.100md.com
References
[1]Gilbert E, et al. The Class II Aminoacyl-tRNA Synthetases and T heir Active Site: Evolutionary Conservation of an ATP Binding Site. J Mol Evol,1 995,40:499.
[2]William HM. Rules that Govern tRNA Identity in Protein Synthesis. J Mol Biol,1993,234:257.
[3]Christian D. The Second Genetic Code. Nature,1988,333:117.
[4]Sun Lai′en, Sun Dongxu, and Zhu Dexi. Molecular Genetics. Nanjing:P ress of Nanjing University,1993.
, 百拇医药
[5]Schimmel P. Aminoacyl tRNA Synthetases: General Scheme of Structure -function Relationship in the Polypeptides and Recognition of Transfer RNAs. Ann Rev Biochem,1987,56:125.
[6]Magaret ES, et al. The Transfer RNA Identity Problem: a Search for R uels. Science, 1994,263:191.
[7]Valery IL. Analysis of Action of the Wobble Adenine on Codon Reading within the Ribosome. J Mol Biol,1995,252:277.
, http://www.100md.com [8]Jennifer N,et al. Changing the Identity of a Transfer RNA. Nature, 1988,321:213.
[9]Valèrie Biou, et al. The 2.9Å Crystal Structure of T. Ther mophilus Seryl-tRNA Synthetases Complexed with tRNASer. Science,1993,263 :1404.
[10]Ya Minghou ,Paul S. A Simple Structural Feature is a Major Determinant of the Identity of a Transfer RNA. Nature,1988,333:140.
[11]Paul S. An Operational RNA Code for Amino Acids and Variatio ns in Critical Nucleotide Sequences in Evolution. J Mol Evol,1995,40:531.
, http://www.100md.com
[12]Akiko S, Ritsuko K, Kazyga N, et al. The Anticodon Loop is a Major Identity Determiannt of Saccharomyces cerevisae tRNALeu, J Mol Biol,1996,263: 707.
[13]Mikio Shimizu. Molecular Basis for the Genetic Code. J Mol Evol,19 82,18:297.
[14]Donald MC. Nucleic Acid Aggregation Geometry and the Possible Evol utionary Origin of Ribosomes and Genetic Code. J Mol Biol, 1982,162:379.
[15]Gutell RR,et al. Identifying Constraints on the Higher-order Str ucture of RNA: Continued Development and Application of Comparative Sequence Ana lysis Methods. Nucleic Acids Research,1993,21:5785.
, http://www.100md.com
[16]Valery IL. Analysis of Action of the Wobble Adenine on Condon R eading within the Ribosome. J Mol Biol,1995,252:277.
[17]Shugo N,Junta D. Dynamics of Transfer RNAs Analyzed by Normal Mode Calculation. Nucleic Acids Research, 1994,22:514.
[18]Christopher Francklyn,Paul Schimmel. Enzymatic Aminoacylation o f an Eight-base-pair Microhelix with Histidine. Proc Natl Acad Sci USA,1990,87 :8655.
, 百拇医药 [19]Theophanides T. Metal Ions in Biological Systems. Int J Quant Chem ,1984,26:933.
[20]Scott JW, et al. A New Force Field for Molecular Mechanical Simulat ion of Nucleic Acids and Proteins. J Am Chem Soc,1984,106:765.
[21]Biosym/Molecular Simulations, Discover2.9.7/95.0/3.0.0, Force Field Simulations. User Guide, Part I. 1995.
[22]Homans SW. A Molecular Mechanical Force Field for the Conformatio nal Analysis of Oligosaccharides: Comparison of Theoretical and Crystal Structur al of Man α1-3 Man β1-4 GlcNAc. Biochemistry,1990,29:9110.
, http://www.100md.com
[23]Liu Ciquan, Wen Yuankai, Jiang Shouping, et al. Quantum Biology and its Applications. Beijing:Higher Education Press,1990.455.
[24]Pack GR,Inter J. Quantum Chem. Quantum Biol Symp,1982,9:81.
[25]William GS,James MB, John RP,et al. Capturing the Structure of a C atalytic RNA Intermediate: the Hammerhead Ribozyme. Science, 1996,274:2065.
[26]Rould MA,Persona JJ,Steitz TA, Structural Basis of Anticodon Loop Recognition by Glutaminyl-trNA Synthetase.Nature,1991,352,213.
(收稿日期 1999-12-05), 百拇医药
单位:姚立新(广东药学院药物研究所,广东广州 510224);曹槐(云南大学现代生物学研究中心);刘次全(中国科学院昆明动物研究所细胞与分子进化重点实验室)
关键词:计算机辅助分子建模(CAMM);识别特性;tRNAHis;GUG;分子力学;氢键网络
广东药学院学报000101 摘 要 本文选用经过实验验证的碱基序列,用简化的方式,构建了被水分子和镁离子修饰的 核酸序列的分子模型,应用分子力学模拟方法对序列进行能量优化,对优化后序列的构象参 数、成键状况和能量数据等进行了分析。对tRNAHHis/GUG的识别特性作了初步的探索 ,得到 了和实验结果相近的结论。此外,还从能力学的角度讨论了溶剂-溶质-溶剂相互作用形成 的网状氢键网络对核酸结构稳定性的影响,探讨了非Crick-Watson G U、U U配对的能力学 特征并存在于被水分子和镁离子修饰的核酸序列中的G U、U U配对情况。
, http://www.100md.com
中图号 P969.1 文献标识码:A 文章编号:1006-8783(2000)01-0001-06
Molecular Mechanics Simulation of Recognition
Identity of tRNAHis/ GUG
YAO Li-xin
(Institute of Materia Medica, Guangdong College of Pharmacy, Guangzhou,510 240)
CAO Huai
(Modern Biological Research Center, Yunnan University)
, 百拇医药
LIU Ci-quan
(Key Laboratory of Cellular and Molecular Evolution, Kunming Institute of Zo ology, Chinese Academy of Sciences)
Abstract A group of nucleic acid sequences retrieved from experiment was analyzed based o n molecular mechanics simulation method to prove tRNA recognition identity that was derived from parts of tRNAHis/GUG sequence. A simplified molecular mod el of nucleic acid sequences with cage-like water molecules and Mg2+ ions was co nstruc ted. Based upon the primary analyses of the optimized molecular models, such as conformation parameters, bonding propensities, and energies of optimized models, etc., the recognition identity of tRNAHis/GUG was probed and the result w as s imilar to experiment-determined ones. Some problems, such as stem-loop structure (hair-pin structure), the impacts of charged metal ions (Mg2+) and H2O molecule s on the stability of nucleic acid sequences, non-Watson-Crick base pairing (i.e . U.U/G.U base pairing), and stabilizing influence of the ″cage-like″ solven t-solvent and solvent-solute bonds were also discussed.
, http://www.100md.com
Key Words computer-aided molecular modeling (CAMM), tRNAHis/GUG , recognition identity, molecular mechanics, hydrogen-bond network
CLC number R 969.1 Document co de:A Article ID:1006-8783(2000)01-0001-06
1 Introduction
The interaction of mRNA with tRNA charged with amino acid takes part in the determination of collinear genetic code. The charging of amino acids with tRNAs is catalyzed by aminoacyl-tRNA synthetases (aaRs)[1].The nucleic acid sequences of tRNAs and minor variations both in nucleic sequ ences and the conformations are concerned with the interaction of tRNAs with ami noacyl-tRNA synthetases, both structurally and functionally[2].So far, 20 kinds of aminoacyl-tRNA synthetases have been reported,each of them responsible for the relevant amino acid and the special tRNA. Usually, each enzym e can be charged with various iso-acceptors of different tRNA molecules[1 ]. Analogous to RNA replicase that has been considered as the first ribosome in the evolution [2], the occurrence of aminoacyl-tRNA synthetases in early protein evolution seems i mproving fidelity of protein synthesis by the special charging with tRNA. Due to the universal conservation of aminoacyl-tRNA synthetases, it has been considere d that the enzymes had come into being in the early evolution, playing the same important role as the catalysis effect that RNAs did in the soup of early organi sm system, the recognition identity between aminoacyl-tRNA and tRNA led to the s peedy evolution of genetic code. This recognition system was called second genet ic code or paracoden[3].
, 百拇医药
The recognition of tRNA is so complex that it is not enough to be determined onl y based on clove structure of tRNA4,5. The explication of recognition identity of tRNAs lies i n the comprehensive studies of tertiary structures of tRNAs and various recognit ion elements in various levels, particularly the involved regions for the recogn ition of aminoacyl-tRNA synthetases and tRNAs. Aminoacyl-tRNA synthetases bind t he inner side of L-shaped tRNAs, the binding locus include accepting arm, DHU-ar m and anticodon arm[6]. Traditional codon-anticodon interactions in ribo somes ar e involved with up-stream factors, down-stream factors and RFs (releasing factor s), while translation process in ribosomes is related with interactions in A-si te, P-site and E-site, etc[7]. While keeping the nucleic acids the same in the a nticodon, mutagenesis of 12 nucleic acids in other regions had changed tRNAl eu t o tRNASer[8]. The 0.29nm crystal structure of T. Thermophilus Seryl-tRNA synthetas es complexed with tRNASer showed that, the anti-parallel super-coiling dom ain in one sub-unit of the aminoacyl-tRNA synthetase contacted with TψC loop and vari able pocket and lead accepting arm of tRNA to the active site of the enzyme [9]. T he recognition identity mainly lay in the contact of backbone of tRNA rather tha n in the sequence identity of tRNA[9]. In vitro nucleic synthesis experi ment als o showed that the changing of charging identity only happened in the mutation of G3.U70 base pair in various mutants of inhibitory E. Coli. tRNAs and relat ive a minoacyl-tRNA synthetases carrying tRNAAla/Glu[10]. Evolutionarily , this nondeg enerate paracodon may be more ancient and more decisive, the charging of amino a cids with tRNAs catalyzed by aminoacyl-tRNA synthetases can be considered as the interactive and indirect charging of two different kinds of genetic languages, and ″entries of genetic″ dictionary are carried by tRNAs and aminoacyl-tRNA sy nt hetases[3]. However, for most tRNAs, the recognition identities are main ly relat ed with nucleic acid sequence of anticodons[2、6、11、12], the clarific ation of this paracodon will contribute to further understanding of the evolution of tran slation process in the transmission of genetic information[6].
, http://www.100md.com
CPK (Corey-Pauling-Koltum) modeling and lock-key relationship has been employe d in the stacking structure of tRNA[13], however, it seems inappropriate to use rigid lock-key relationship to describe the flexible interaction of nuc leic acid with proteins. Various methods have been employed in the simulation or theoretical calculation of the recognition identity of tRNA[14、15].Us ing simp lified method to analyze may be possible, such as energy minimization and normal mode methods[16、17]. Based on four sequence retrieved from the concern ed experi ment[18], computer-aided molecular modeling, molecular mechanics methods were performed in this study by using simplified methods.2 Computational Methods
, http://www.100md.com
All the modeling, visualization, simulation, and analyses in this study were done using Biosym/Molecular Simulation Insight-Ⅱ software in SiliconGr aphics Indigo2 workstation (Mountain View, CA 94043-1389). The measuremen t of dihedral angles of the sequences was conducted with Tripos Sybyl molecular modeling software.
3 Molecular Modeling
The stem region of the retrieved[18]sequences was constructed as A-RNA d uplex, and the loop region was constructed as single A-RNA helix. Consideri ng there are Mg2+ ions and H2O molecules in the physiological environmen ts of tRNA, molecular models Mg2+ and H2O molecul es were constructed, while neuridine molecules and sodium ions which usually bin d to tRNAs were omitted due to the restriction of the processing capacity of the software package used. The interaction models of metal ions (Mg2+) and H 2O molecule s with single nucleic acids of A, U, G and C[19] gotten from Laser-Rama n spectroscopy were constructed and then employed in the construction of the nuc leic acid chains (See Fig. 1). The cap of metal ions and water molecules were th en merged or docked to nucleic acid chains (See Fig. 2) separately.
, http://www.100md.com
4 Force Field Selection
ESFF force field from Discover 2.9.7/95.0/3.0.0 was selected in the simulati on. The atomic parameters used in this force field were obtained from experiment s instead of adaptation of ab initio calculation and experimental data[20]. The coverage of ESFF force field is particularly useful for the simulation of o rganic compounds and organi c-metal mixtures [21].
, 百拇医药
Fig. 1 Model of the active interaction between positive ion Mg( H2O)62+and N7 of purines. (From T. Theophanides, 1984) (Hydrogen bonds are showed with dashed lin es)
Fig. 2 Secondary structure of E. coli (G1.C73)tRNAHis/GUG([a]), (G 1.C33)Miniheli xAla([b]), (G1.C23)MicrohelixAla([c]), MinihelixHis([d ]) and ( G1.C73)MicrohelixHis([e]) (from Christopher Francklyn and Paul Schimmel , 1990)
, http://www.100md.com
5 Energy Calculation and Structure Optimization
After the construction of initial structures of complexes with Mg2+ ions and H2O molecules, the whole structures were th en refined and minimized by using steepest descent, followed by conjugate gradie nt, Newton-Rapson, and iterative Newton-Rapson methods automatically and alterna tively till minimum energies were reached. The energy was convergent and root-me an-square (rms) energy gradient of 0.01 kcal/mol ?2 was reached. The de fault charg es from Discover 2.9.7/95.0/3.0.0 package were employed throughout. Four con trol s with the nucleic chains of the same nucleic acid sequences without Mg2+ ions and H2O molecules were also optimized under the same force field and same conditi ons.
, 百拇医药
6 Result and Discussion
6.1 Energy Optimization Seven energy terms were obtained after energy optimization (See table 1).
Tab. 1 Energy terms of the sequences after energy optimizatio n kcal/mol
Total potentialenergy
Internal
Bond
Angle
Torsion
, http://www.100md.com
Out-of
-p lane
Non-
bond
Vdw
vdw-
repulsive
vdw-
dispersive
Electrostati c
MinihelixHis
571.64
, http://www.100md.com
1139.54
108 .09
6 94.87
318.85
17.74
-567.90
576.21
4129.72
-3553.52
-1155.11
MicroehlixHis
-204.07
, 百拇医药
814.77
73.95
486.63
240.01
14.18
1018.84
418.73
3170.23
-2571.50
-1437.57
MinihelixAla
12.42
, 百拇医药
1091.37
96.74
670.84
310.65
13. 14
-1078.95
536.82
4068.25
-3531.43
-1615.78
MicrohelixHis
-837.49
, 百拇医药
774.46
64.74
470.77
226.63
1 2.31
-1611.94
366.55
3098.58
-2732.03
-1978.49
Control.
4891.14
, 百拇医药
778.63
44
443.73
27.172
18.87
4112.4 9
41.77
1771.48
-1729.71
4070.72
.Note: the control sequence was the same as that of MicrohelixAla, but without Mg2+ ions and H2O molecules.
, http://www.100md.com
Analyzed energetically,it is reasonable that the optimum energies of Mini helixHis and MinihelixAla were larger than those of MicrohelixHi s and Microehli xAla. Although the system of sequ ences with Mg2+ ions and H2O molecules were much more complex than those without Mg2+ ions and H2O molecules, the energy of the sequences with Mg2+ ions and H2O molecules were much lower than those of sequences without Mg2+ ions and H 2O mol ecules, partially due to the forming of cage-like solvent-solvent and solvent-so lute hydrogen-bond network and interaction with metal ions. The visualization in the optimization process also proved,the nucleic acid chains without Mg2+ ions and H2O molecules di sbanded much easier. The transitory ″cage-like″ hydrogen bond network between solvent- so lvent and solvent-solute bonds can provide extra stationary factors and reduce t orsion resonance between glycosidic bonds[22], similar to the polyelectr olytic c haracteristics of DNA under ionic environment, and the improvement of dissociati on temperature of DNA caused by the increasing of positive metal ions since meta l io ns had stabilizing effects on nucleic acid molecules[23]. After energy optimiza tion, the Mg2+ ions were mainly distributed in the groove of the nucleic a cid seq uences, in accordance with high salt model (Pack, 1982)[24] and similar to the ion distribution in rRNA[25].
, 百拇医药
It′s noticed that there were G.U and U.U wobble base-pairs (or non-Watson-C r ick base pairs) in MinihelixHis and MinihelixAla sequences. We calcu lated the G.U and U.U base pairs with molecular mechanics using Amber force fi eld, it was found that the increasing variation of hydrogen bond energy term of U.U base pair is larger than that of G.U base pair. Since Hoogsteen hydrogen b onds could be formed between wobble base pairs, the hydrogen bond energy became larger than those of Watson-Crick hydrogen-bonds, while the propensity of U.U b ase pair was smaller than that of G.U base pair. Observed in the final structur e after optimization, there was no hydrogen bond between two wobble uracil pyrom idines, however, there were H3-O2 hydrogen bonds which formed pyromidine rin gs in the U.U wobble pairing. There were also some changes in the hyd rogen bonds close to the wobble pairs. No hydrogen bond was formed in U.A p air above the U.U wobble pairing, while hydrogen bonds different from Watson-Cr i ck pairing appeared in G.C pair that was just below U.U pair. The plane betwee n purine and pyromidine rings was twisted compared with that in Watson-Crick pair. (G)N4-H…N3(C) hydrogen bond was formed in the G C pair. The hydrogen bond bet ween purine ring of G and pyromidine of C, (G)N1-H1…O3(C) was different w ith t hose formed in normal Watson-Crick pairs, i.e. (G)N1-H1…O3(C), (G)N1-H …N3(C) and (G) N2-H…O2(C).
, 百拇医药
In addition, the co-planety in G.U was also poor without hydrogen bond betwee n them, while (G)N3-H2…O2(C), (G)N1-H1…N3(C) hydrogen bonds were fo rmed in G.C pair just above G.U wobble pair. Between G in G.U wobble pair and G in G.C pa ir just below the wobble one, (G in the wobble pair) N2-H…O6(C) hydrogen bo nd was formed. Hydrogen bonds different from Watson-Crick pair, i.e. (G)O6-H…N 3(C ) and (G)N1-H…N3(C) hydrogen bonds were formed in G.C pair just below G.U wobble pair.
, http://www.100md.com
The dihedral angles of the four optimized nucleic acid chains were measured (See table 2.). It can be concluded that the charging of four nucleic acid cha ins (i.e. MinihelixHis, MicrohelixHis, MinihelixAla and Microh elixAla) with amin oacyl-tRNA synthetase is not determined by the whole conformation of the nucleic chains,it is closely related with conformations in some special regions since it seems that no similarity exist between the four nucleic acid chains ju dged only from measured results.
, 百拇医药
Tab. 2 Average diheral angles of the four optimized
nucleic acid chains
MinihelixHis
MicrohelixHis
MinihelixAla
Microheli xAla
α
186.25
227.47
, 百拇医药 -157.28
-119.49
β
-181.75
182.65
-175.37
-179.02
γ
150.14
-242.31
-107.79
111.04
δ
, 百拇医药
89.78
87.91
82.11
74.35
ε
-162.87
-158.58
-177.91
-152.02
ζ
-85.58
-80.84
-68.47
, 百拇医药
-75.29
χ
-131.60
-139.79
218.06
210.39
υ0
-7.92
-0.58
-8.56
0.61
υ1
-7.94
, 百拇医药
-15.33
-9.01
-22.57
υ2
19.37
24.35
21.58
34.4
All of the four sequences in this simulation were composed of stem-loop or hairpin structure. It has been proposed that loop structure p lays an important role in the process of genetic information flow[4]. The seq uences in the stem regions of MinihelixAla and MicrohelixAla were no t the same , however, the average dihedral angles in loop regions of the two sequences mea sured were almost equivalent (See Table 3 and Table 4).Tab. 3 Average angles in the stem regions of
, 百拇医药
the fo ur optimized nucleic acid chains
MinihelixHis
MicrohelixHis
MinihelixAla
Microheli xHis
α
194.73
24.361
-142.82
-105.48
, 百拇医药
β
-176.33
182.67
-175.57
-179.69
γ
148.25
-249.61
-99.36
101.69
δ
93.09
91.59
, 百拇医药
84.59
79.15
ε
-162.55
-153.44
-176.14
-150.11
ξ
-95.14
-86.76
-71.29
-80.67
χ
, 百拇医药
-128.78
-142.01
205.44
210.50
υ0
-4.75
-0.61
-8.40
-1.49
υ1
-8.75
-12.98
-9.25
, 百拇医药
-19.87
υ2
17.60
20.74
21.85
32.15
The conservative G1.C73 base pair had been proved necessary experimentally in the charging with aminoacyl-tRNA synthetase and tRNAAla. No Watson-Crick h ydroge n bond formed between G1.C33, G1.C23 base pairs in MinihelixHis (G1.C33 ), Microh elixHis (G1.C23), MinihelixAla (G1.C33), and MicrohelixAla ( G1.C23)(see Table 5),the same as that in C1.G73 base pair in tRNAAla of Saccaromyces cerevisiae from Brookhaven Protein Databank (PDB). While a non-Watson-Cr ick hydrogen bond w as formed between G1.C73 base pair, some hydrogen bonds were formed between non-pairing bases. The result is in accordance with what has been proved experim entally. And the 2.8 X-ray crystalloid structure of tertiary complexes of tRNAGln, aminoacyl-tRNA synthetase and ATP had proved that the structur e of tRNA in c omplexes was not the same as that of free tRNA, where hydrogen bond between U1 A72 base pair was broken, and a new hairpin structure was formed in CCA of 3′- e nd, which was sunken into a pocket of the enzyme[26]. It seems that non- Watson-C rick hydrogen bonds in G1.C33 and G1.23 base pairs are unstable, and thus lik ely to be broken.Tab. 4 Average angles in the stem regions of
, 百拇医药
the four optimized nucleic acid chains
MinihelixHis
MicrohelixHis
MinihelixAla
Microh elixHis
α
175.90
151.60
188.17
188.17
, http://www.100md.com
β
-177.39
159.26
182.71
182.78
γ
137.51
146.30
136.23
136.42
δ
77.93
78.67
, 百拇医药
59.79
59.69
ε
190.58
190.82
-162.72
-162.79
ζ
-50.47
-46.08
-60.75
-60.34
χ
, http://www.100md.com
-146.81
-138.17
-148.25
-148.26
υ0
-0.48
-14.66
6.45
6.31
υ1
-21.70
-9.32
-29.97
, http://www.100md.com
-29.90
υ2
34.13
27.18
40.55
40.56
Tab. 5 Hydrogen bonds in conservative G1.C23 and G1.C33 base pairs in MinihelixHis,MicrohelixHis, MinihelixAla, and MicrohelixAla.
Hydrogen bonds formed among G1 C23 or G1 C33 base pairs and nearby bases
, http://www.100md.com
MinihelixHis
No hydrogen bond formed in G1 C33 bas e pair and the plane in G1 C33 base pair was twisted
MicrohelixHis
(G1)O6…H-N4(C23) hydrogen bond was formed; and (G2)N3…H-N4(C22) and (G1)N2…H-N4(C23) hy drogen bonds were form ed between G1 and C72 bases
, http://www.100md.com
MinihelixAla
No hydrogen bond formed in G1 C33 base pair and the plane in G1 C33 base pair was twisted
MicrohelixAla
(G1)O6…H-N4(C33) hydrogen bond bet ween G1.C33 base pair and (G2)N2-H…O2(C33) hydrogen bond between G2.C33 base pair were formed.
, http://www.100md.com
References
[1]Gilbert E, et al. The Class II Aminoacyl-tRNA Synthetases and T heir Active Site: Evolutionary Conservation of an ATP Binding Site. J Mol Evol,1 995,40:499.
[2]William HM. Rules that Govern tRNA Identity in Protein Synthesis. J Mol Biol,1993,234:257.
[3]Christian D. The Second Genetic Code. Nature,1988,333:117.
[4]Sun Lai′en, Sun Dongxu, and Zhu Dexi. Molecular Genetics. Nanjing:P ress of Nanjing University,1993.
, 百拇医药
[5]Schimmel P. Aminoacyl tRNA Synthetases: General Scheme of Structure -function Relationship in the Polypeptides and Recognition of Transfer RNAs. Ann Rev Biochem,1987,56:125.
[6]Magaret ES, et al. The Transfer RNA Identity Problem: a Search for R uels. Science, 1994,263:191.
[7]Valery IL. Analysis of Action of the Wobble Adenine on Codon Reading within the Ribosome. J Mol Biol,1995,252:277.
, http://www.100md.com [8]Jennifer N,et al. Changing the Identity of a Transfer RNA. Nature, 1988,321:213.
[9]Valèrie Biou, et al. The 2.9Å Crystal Structure of T. Ther mophilus Seryl-tRNA Synthetases Complexed with tRNASer. Science,1993,263 :1404.
[10]Ya Minghou ,Paul S. A Simple Structural Feature is a Major Determinant of the Identity of a Transfer RNA. Nature,1988,333:140.
[11]Paul S. An Operational RNA Code for Amino Acids and Variatio ns in Critical Nucleotide Sequences in Evolution. J Mol Evol,1995,40:531.
, http://www.100md.com
[12]Akiko S, Ritsuko K, Kazyga N, et al. The Anticodon Loop is a Major Identity Determiannt of Saccharomyces cerevisae tRNALeu, J Mol Biol,1996,263: 707.
[13]Mikio Shimizu. Molecular Basis for the Genetic Code. J Mol Evol,19 82,18:297.
[14]Donald MC. Nucleic Acid Aggregation Geometry and the Possible Evol utionary Origin of Ribosomes and Genetic Code. J Mol Biol, 1982,162:379.
[15]Gutell RR,et al. Identifying Constraints on the Higher-order Str ucture of RNA: Continued Development and Application of Comparative Sequence Ana lysis Methods. Nucleic Acids Research,1993,21:5785.
, http://www.100md.com
[16]Valery IL. Analysis of Action of the Wobble Adenine on Condon R eading within the Ribosome. J Mol Biol,1995,252:277.
[17]Shugo N,Junta D. Dynamics of Transfer RNAs Analyzed by Normal Mode Calculation. Nucleic Acids Research, 1994,22:514.
[18]Christopher Francklyn,Paul Schimmel. Enzymatic Aminoacylation o f an Eight-base-pair Microhelix with Histidine. Proc Natl Acad Sci USA,1990,87 :8655.
, 百拇医药 [19]Theophanides T. Metal Ions in Biological Systems. Int J Quant Chem ,1984,26:933.
[20]Scott JW, et al. A New Force Field for Molecular Mechanical Simulat ion of Nucleic Acids and Proteins. J Am Chem Soc,1984,106:765.
[21]Biosym/Molecular Simulations, Discover2.9.7/95.0/3.0.0, Force Field Simulations. User Guide, Part I. 1995.
[22]Homans SW. A Molecular Mechanical Force Field for the Conformatio nal Analysis of Oligosaccharides: Comparison of Theoretical and Crystal Structur al of Man α1-3 Man β1-4 GlcNAc. Biochemistry,1990,29:9110.
, http://www.100md.com
[23]Liu Ciquan, Wen Yuankai, Jiang Shouping, et al. Quantum Biology and its Applications. Beijing:Higher Education Press,1990.455.
[24]Pack GR,Inter J. Quantum Chem. Quantum Biol Symp,1982,9:81.
[25]William GS,James MB, John RP,et al. Capturing the Structure of a C atalytic RNA Intermediate: the Hammerhead Ribozyme. Science, 1996,274:2065.
[26]Rould MA,Persona JJ,Steitz TA, Structural Basis of Anticodon Loop Recognition by Glutaminyl-trNA Synthetase.Nature,1991,352,213.
(收稿日期 1999-12-05), 百拇医药